The landslide in my upper photo was triggered by a magnitude 7.1 earthquake on 5 May 1968, shaking weathered granite and greywacke in heavily forested slopes in the northwest part of the South Island of New Zealand. It dammed the Buller River and created a 7-km-long lake. Fortunately, the landslide dam did not break catastrophically, but gradually, so the downstream town of Westport was not inundated. The quake caused extensive damage and killed three people.
In 1929, the nearby magnitude 7.7 Murchison quake and a M 6.2 aftershock killed 17, mostly from landslides that destroyed homes. The fault rupture in my lower photo is marked by a sign, but it is so overgrown with vegetation you’d hardly notice the scarp. This part of New Zealand is lush rain forest. My two photos were taken in February 1981.
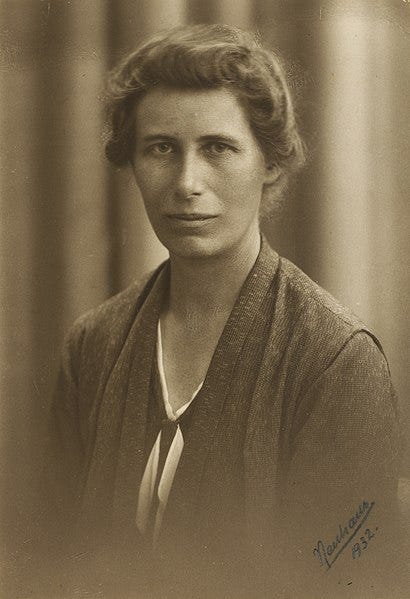
The 1929 quake was the source of the data that Danish seismologist Inge Lehmann used in 1936 to demonstrate that the earth must have a solid inner core, not just a homogenous liquid core. She was born May 13, 1888, and died in 1993 at age 104.
Lehmann recognized that certain seismic waves that traveled through the earth’s mantle and core were delayed in their arrival times by an amount that could not be explained by a simple, uniform core, and furthermore, some of the arrivals were offset from their expected locations. That led her to infer the existence of a liquid outer core as well as a solid inner core, transmitting seismic energy at different rates and for some of it, bouncing off or refracting through the inner-outer core contact. Lehmann determined that the inner core had a radius of about 1,400 km (870 miles), remarkably close (given the tools and data of 1936) to the modern accepted value of 1,221 km (759 mi).
Both the 1968 and 1929 earthquakes resulted from strike-slip motion on splays of the Alpine Fault, a major transform fault similar to the San Andreas in California. The movement on the Alpine Fault is ultimately a consequence of oblique motion between the Australian Plate and the Pacific Plate, ripping the largely submerged continent of Zealandia apart.
Analysis suggests that the hill slopes that failed in 1968 (magnitude 7.1) were influenced by previous damage incurred in the M 7.7 1929 earthquake in the same area. That’s not exactly surprising, but it is nice to quantify it. The epicenters of the two quakes were about 21 km apart but faults that broke the surface were as close to each other as 7 km. (“Spatial distributions of earthquake-induced landslides and hillslope preconditioning in the northwest South Island, New Zealand,” Parker and others, 2015, Earth Surface Dynamics 3, p. 501.)
For more about the tectonics of New Zealand, please visit my History of the Earth post on Zealandia.
I don’t have any specimens from the Earth’s core (no one does). The closest I can come may be this nugget of josephinite, a local (Josephine and Jackson Counties, Oregon, USA; it is one of Oregon’s state minerals) name for rocks composed mostly of awaruite, a natural nickel-iron alloy. In the 1970s josephinite was argued to be from the lower mantle, the core-mantle boundary, or possibly from the core itself (e.g., Bird & Weathers, 1979, Origin of josephinite, Geochemical Journal, 13(2), p. 41-55; Dick & Gillete, 1976, Josephinite: specimens from the earth’s core? – a discussion: Earth & Planetary Science Letters, 31:2, p. 308-311).
The Klamath Mountains of southern Oregon where the josephinite is found have definitely brought rocks, ranging from oceanic crust to mantle-sourced material, to the surface. As far as I can tell, the consensus seems to be that the josephinite is related to alteration and mineralization of the serpentine in which it is found, and that it developed relatively near the surface (Staudacher and Allègre, 1990, The origin of josephinites: A new noble gas study: Earth & Planetary Science Letters, 98:3-4, p. 380-389). But it also seems that controversy continues.
The type locality for awaruite is the Gorge River, New Zealand, about 350 km from the site of the earthquakes Inge Lehmann used to recognize the earth’s core. The mineral was named for the Awarua River, mistaken as the site where it was found in 1885.
Another example of mantle-derived material is the two xenoliths of olivine (probably the forsterite end member, Mg2SiO4) in the specimens above. A xenolith (“strange rock”) is a piece of the surrounding country rock ripped off by and into molten material rising in intrusive bodies like plugs, dikes, sills, and batholiths, not melted and incorporated into the rock but retaining some or much of its original mineralogy.
There are many locations worldwide for olivine xenoliths like this, and I don’t know for sure where mine are from, but the most likely location is San Carlos, Gila County, Arizona USA, where basaltic volcanic eruptions brought the olivine to the surface quite recently, about 4.2 to 1.0 million years ago (Wood and Kienle (eds), 1990: Volcanoes of North America: Cambridge Univ. Press). It’s quite a small volcanic field, but it must have tapped deep sources to pass through the mantle to pick up the fragments of the material it incorporated as xenoliths. It lies approximately along the southwestern edge of the Colorado Plateau, a dramatic change in crustal thickness and rock type, and (as with multiple places around the Colorado Plateau) a good location for magmatic “leaking” that produced volcanic fields.
If you are interested in my other posts related to the History of Geology, please see this post with a downloadable PDF.
Before I had even read the text, I thought "That looks like the slip near Lyell in New Zealand". Unmistakeable once you have seen it yourself!
(ps: "caption..." 😁)
Have you ever gone Rockhounding on a landslide and found minerals?